-
Addressing the root cause of rare diseases — the promise of antisense oligonucleotides
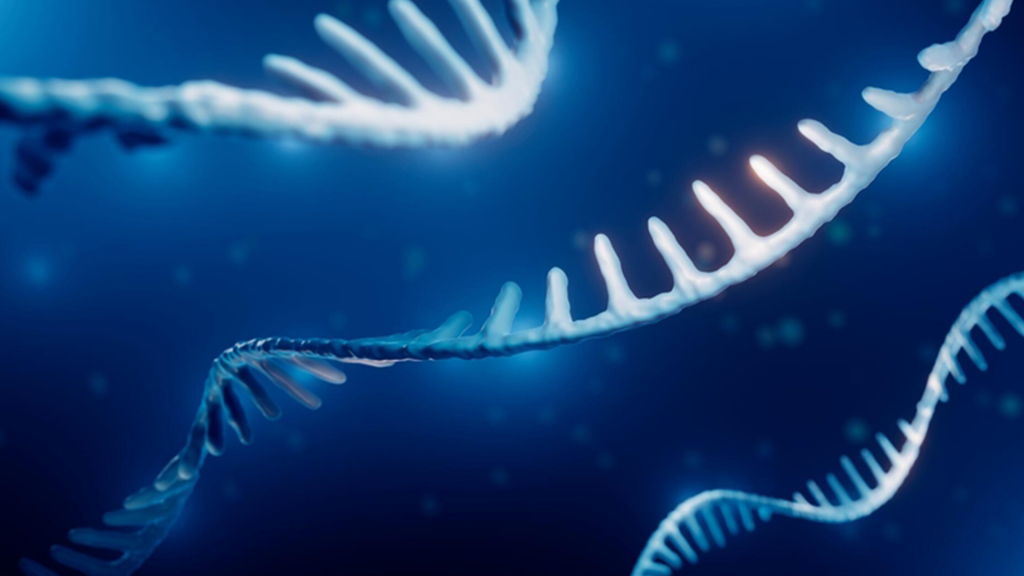
The central dogma of molecular biology — cited in countless science textbooks — states that DNA is transcribed into RNA, and RNA is translated into proteins. This basic idea is key to understanding how genes are expressed and how to overcome genetic defects that cause disease.
For much of history, researchers have treated diseases by targeting the proteins involved, such as giving insulin for diabetes or statins for heart disease. Over the last couple of decades, however, they have pursued gene therapies that target disease at the level of the DNA, including developing drugs that correct the genetic defect in sickle-cell disease. More recently, researchers have been advancing treatments that go after the often-overlooked molecule RNA. To do so, many are harnessing a technology known as antisense oligonucleotides.
RNA molecules act as intermediaries between DNA and protein, but they also do so much more, says Margot Cousin, Ph.D., a translational genomics scientist at Mayo Clinic. There are RNAs that can perform biochemical reactions, and others that can regulate gene expression.
"We're trying to use some of the processes that exist in our body to design these drugs and make them work for us," says Cousin.
Antisense oligonucleotides, or ASOs, are short, synthetic strands of nucleotides designed to bind to complementary RNA sequences like the teeth in a zipper. These ASOs can look and behave differently depending on the type of genetic defect they are designed to target. An estimated 15 to 30 percent of genetic diseases are caused by mutations that disrupt RNA splicing, the process by which stretches of noncoding RNA are removed and the remaining coding regions are spliced back together.
"It's essentially like putting all the beads that you need to make your protein together and getting rid of all the string in between that you don't need," says Dr. Cousin.
Splicing mutations can cause these beads to be put together incorrectly, producing defunct proteins. Dr. Cousin says that researchers can create ASOs to block these mutations and set splicing back on track. For example, the first FDA-approved treatment for spinal muscular atrophy (SMA) is an ASO that modifies the splicing of the SMN2 gene to ramp up the production of functional SMN protein, which is lacking in SMA patients.
Alternatively, mutations can cause a gene to be expressed abnormally or to acquire new properties. In those cases, scientists can design ASOs to specifically bind to the RNA sequences generated by the faulty genes and flag them to be degraded, effectively reducing the production of harmful proteins.
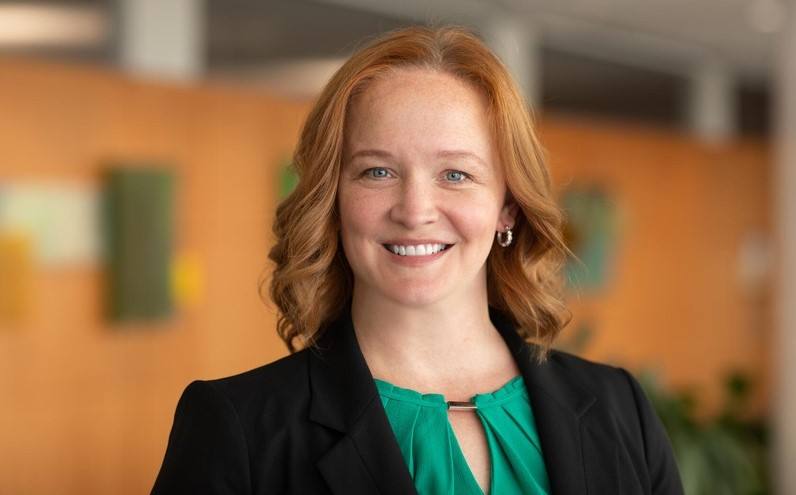
Dr. Cousin and her colleagues are currently assessing this technology for what is known as n=1 cases — those patients with ultra-rare diseases who have no other treatment options.
"These are patients with severe or life-threatening conditions where we know the risk of not treating is greater than the risk of treating with an experimental ASO," she says. "The chemistry of ASOs have already been approved for medicinal use, and their modular nature means that the researchers can simply change the order of the nucleotides to reach a specific target for patients who may benefit from this strategy."
"Our understanding of the human genome and genetic causes of disease continues to grow and therapeutic tools like ASOs are increasingly being pursued to overcome them," said Dr. Cousin. "The study and development of genetic therapies including ASOs will bring precision medicine to those in need with increasing frequency, making them a remarkable drug tool to explore right now."
Related Articles
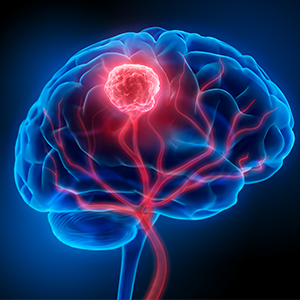
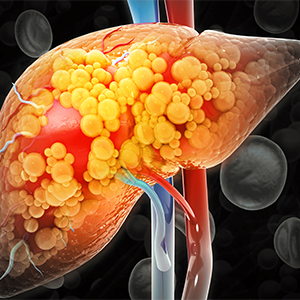
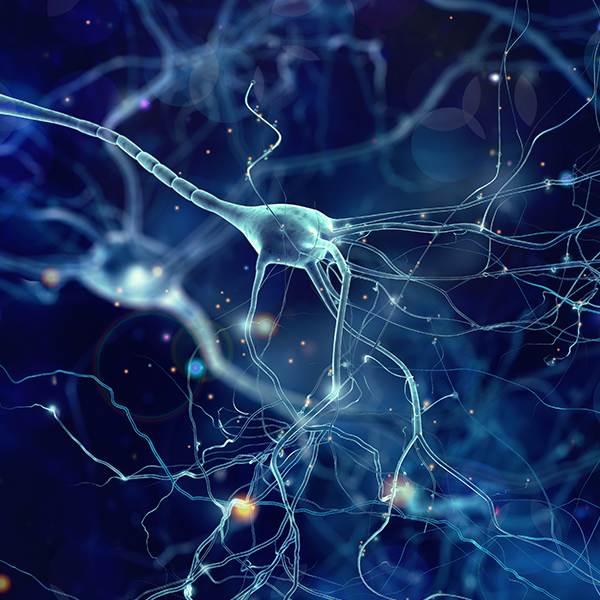